Missions
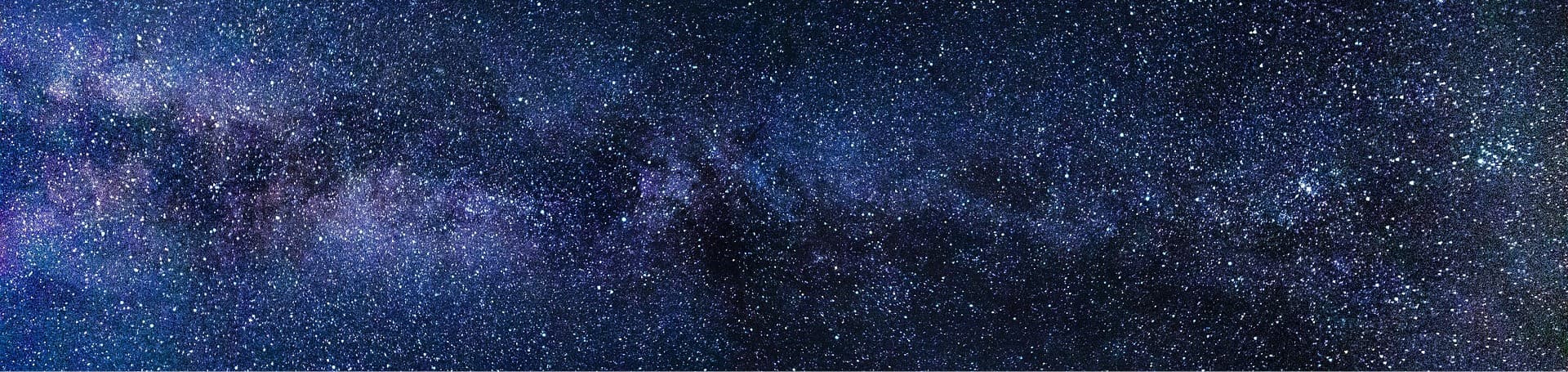
Scientific Enigmas for XRISM
XRISM casts its gaze upon stars, galaxies, and the ethereal "plasma" weaving through them, unraveling the intricate tale of the universe's grand tapestry. Like our Milky Way, galaxies house 100 billion sun-like stars, forming cosmic constellations. The universe itself is a vast archipelago, with clusters of galaxies as its colossal islands.
In the cosmos bathed in visible light, galaxies appear as scattered islands with apparent emptiness in between. Yet, beneath this cosmic facade, XRISM's X-ray vision reveals a hidden narrative—a sizzling "ocean" of high-temperature plasma connecting galaxies. This plasma, an ionized gas born of cosmic heat, dominates the mass of galaxy clusters, playing a central role in the cosmic ballet. XRISM, armed with an ultra-high-resolution X-ray spectroscopic imager, explores this cosmic sea, measuring elements, temperature, density, and velocity with unprecedented precision.
Picture rivers of plasma circulating among stars and galaxies, akin to rivers, oceans, clouds, and rain on Earth. XRISM, with its X-ray microcalorimeter, unveils the universe's secrets with unparalleled precision, a tool finely tuned to explore the magnificent cosmic mysteries.
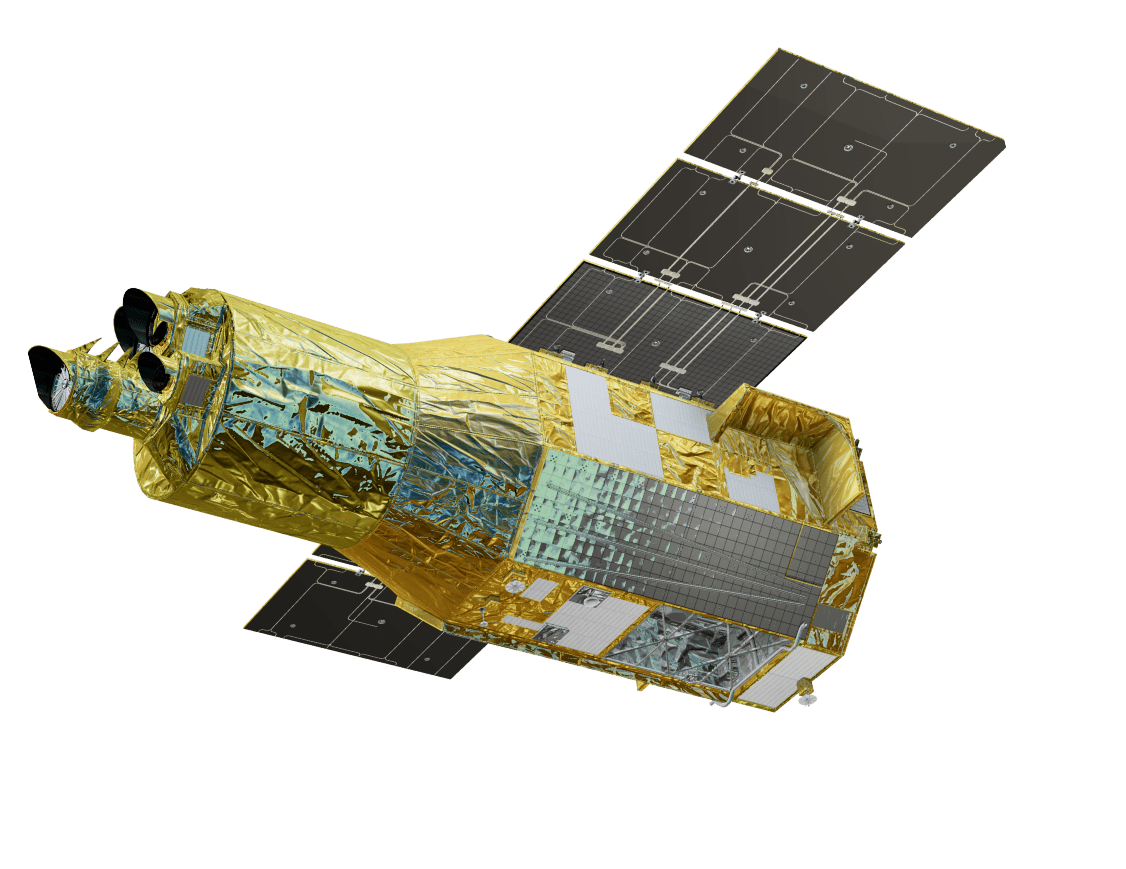
Enigmas1The Assembly of
Clusters of Galaxies
A cluster of galaxies, the largest structure in the universe, is formed with a balance between the immense gravitational pull and the outward pressure of the hot plasma. X-ray observations before XRISM could only measure the temperature, and thus the thermal pressure, of the plasma, so we couldn't fully understand how the total gas pressure balances with the gravitational pull in our observational results. The new X-ray imaging spectrometer onboard XRISM is able to measure the plasma wind velocity to reveal its dynamical pressure. By measuring both the thermal and dynamical pressure of plasmas, XRISM will measure all aspects of this balancing act, revealing the "mechanical design drawing" of the formation of clusters of galaxies.
How were "clusters of galaxies" formed?
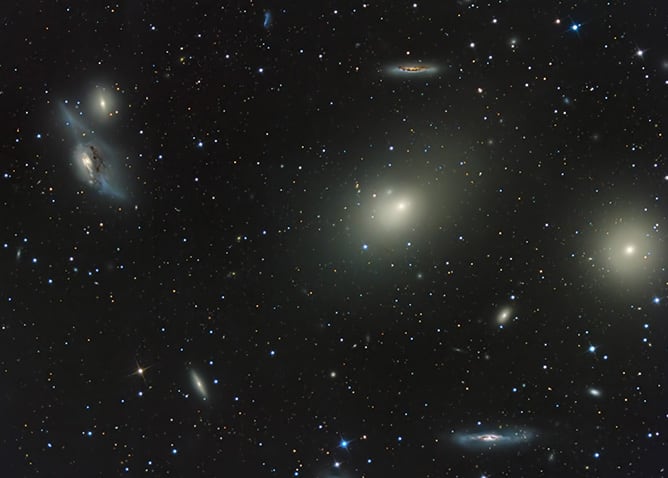
The structure of clusters of galaxies are apparently formed under static equipartition between the pressure of hot plasma and the gravity caused mainly by dark matter. This seems to be a strange problem in kinematics. Because it radiates X-rays, the hot plasma is gradually cooled. The cooled plasma is getting denser in the central region, and is thus more effective in radiation and cooling. Since it makes the plasma cooler, this is a positive feedback process. Thus, the hot plasma in clusters of galaxies are expected to cause gravitational core corruption in 100 million to 1 billion years. However, clusters of galaxies are stable for 10 billion years, and we see no sign of corruption.
So, what in the cluster of galaxies compensates for the inevitable energy loss and prevents this corruption? Candidates proposed are heat conductance from the core surrounding hot plasma, dissipation of kinetic energy of galaxies, or heating by hot outflow plasma from the central supermassive black hole (active galactic nucleus). To solve this problem, the key is temperature and velocity of the hot plasma. The high resolution X-ray spectroscopy provided by XRISM will enable us to determine the temperature and velocity distribution in the plasma of a cluster of galaxies, and leads us to understand the hidden dark matter distribution.
Enigmas2The Chemical Makeup
of the Universe
Until 300,000 years after the birth of the universe, only four elements from the periodic table existed. The universe was flavorless, as many of the indispensible elements for our life, like carbon, oxygen, or other metals were produced in the life of stars born after. These elements produced in stars were distributed amongst the galaxies, and flavored the stars, planets, and life among them.The history of enrichment is called the "chemical evolution" of the universe. The X-ray imaging spectrometer onboard XRISM detects these elements with unprecedented sensitivity and measures their velocity in space. Thus, XRISM reveals a kind of recipe for the flavor of the universe.
How does the universe produce and distribute elements?
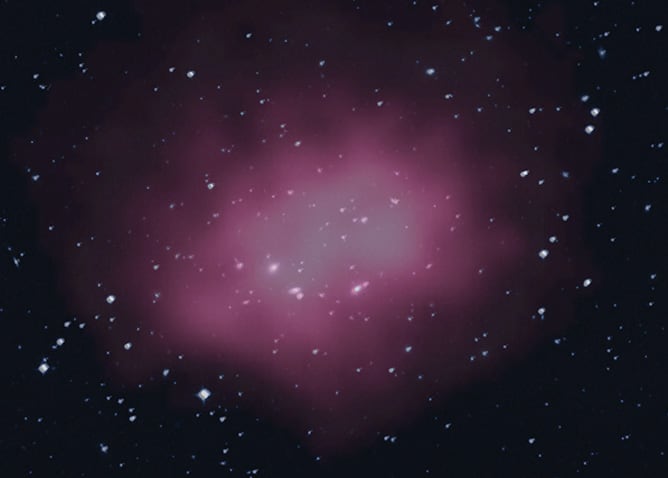
The history of nucleosynthesis --- production of elements --- is recorded in the hot plasma of clusters of galaxies. Elements produced in stars and supernovae were gradually distributed and enriched this intergalactic plasma. The abundance patterns of various elements depends on the distribution of mass stars and the types of supernovae in the universe.
The knowledge of this abundance pattern is obtained through observation of stars, interstellar plasmas or supernova remnants in our Galaxy. With the XRISM high resolution X-ray spectrometer, we can detect rare elements that are thus far overlooked and measure their dissipation velocity.
As with rivers, seas, or atmosphere on the Earth, the hot plasma carries and circulates materials in the universe. The materials dissipate in interstellar space, and are recycled for stars and planets of the next generation or distributed into intergalactic space. With these flows, the circulation of elements is a dynamic process.
Clues to the "missing baryon problem"
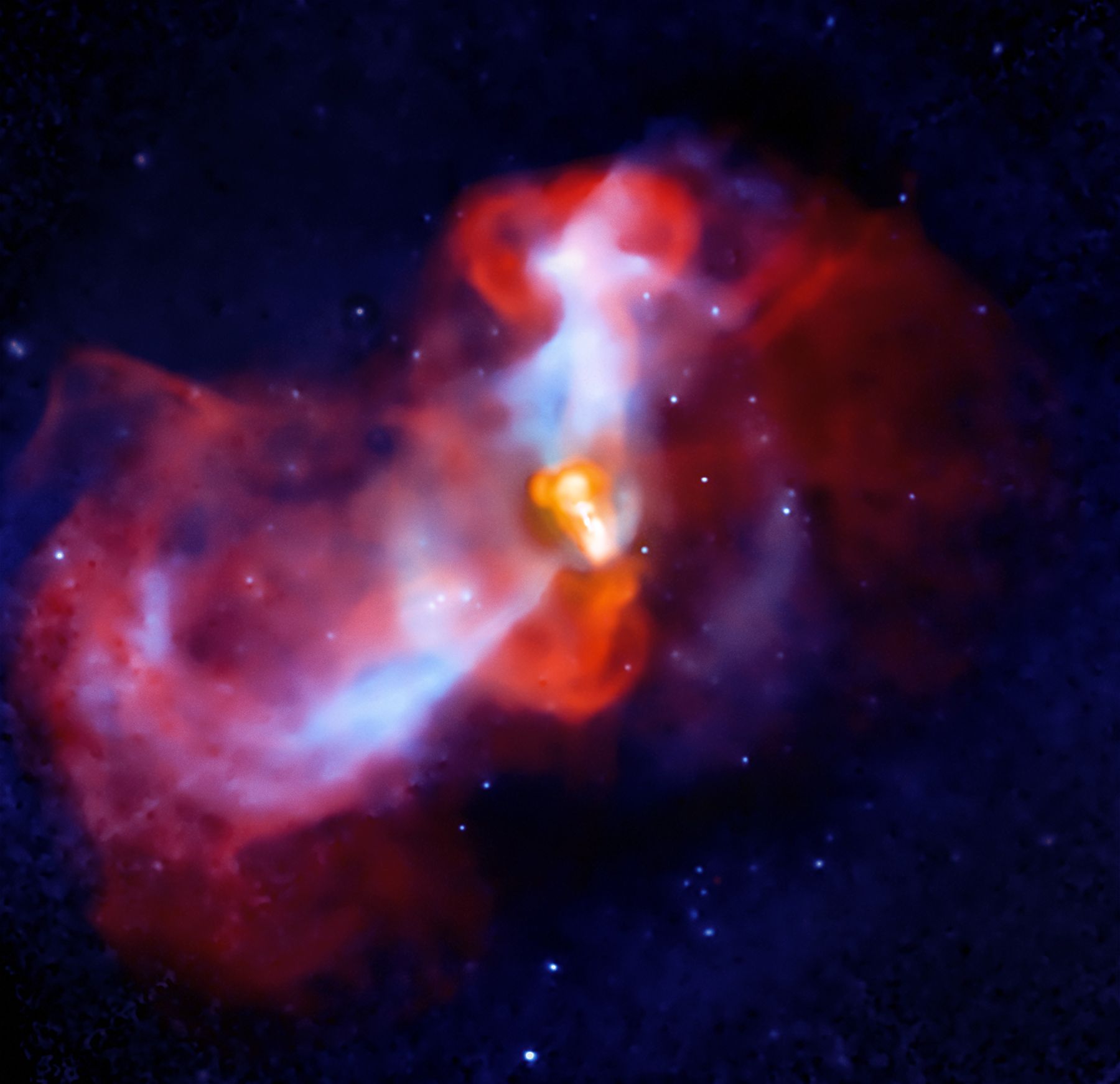
The dominant components of the hot plasma mass are protons and neutrons, two baryons that play important roles in the material history of the universe. However, these baryons were not always "hot". When the first baryons were produced in the young hot universe, they were fully ionized. As the universe expands and is getting cooler, those ionized atomic nuclei captured electrons to become neutral atoms in about 300,000 years. After 300,000,000 years, the first of generation stars were born, and their radiation heated and re-ionized the atoms to produce hot plasmas.
Although we observe this hot plasma in the nearby universe, we hardly observe the "warm" plasma during the age of re-ionization. Neutral or low temperature gas is observed with radio waves or optical light. We observe the hot plasma in X-ray band. However, the rest of the "warm" plasma ---- of 10,000 to a few 1,000,000 Kelvin is difficult to observe. These warm plasmas emit ultraviolet (UV) radiation. It is difficult to observe UV radiation from large distances, since UV radiation is easily absorbed by the large amount of hydrogen gas in the universe. We call those expected but not observed warm plasmas "missing baryons". The amount and element abundance of the missing baryons is an important piece to complete the puzzle of material history in the universe.
XRISM is able to observe the warm plasmas, although it cannot observe UV. With a prism, you may have observed dark lines in the spectrum of light from the Sun. These absorption lines are caused by the relatively low temperature chromosphere above the sun photosphere. With XRISM, we are able to observe the warm plasma through absorption features in the background X-ray source spectrum. We use distant but bright active galactic nuclei, for example, as the background light. The precise spectroscopy of XRISM is essential to determine the warm plasma's temperature and elemental abundance.
Enigmas3The Extremes of Spacetime
There are places that no spaceship can ever take us. One is the distant universe running away from us due to the expansion of the universe, so fast we can never catch up. Another is the inside of a black hole. Black holes distort the surrounding spacetime and expands the wavelengths of X-ray photons from the nearby plasma. The X-ray imaging spectrometer onboard XRISM measures wavelength precisely, and will reveal these distortions of spacetime and the motion of material surrounding a black hole. This information enables us to investigate the spacetime around black holes, as well as the inflow and outflow of matter and energy from the black hole's surroundings.
Structure of plasma surrounding compact objects
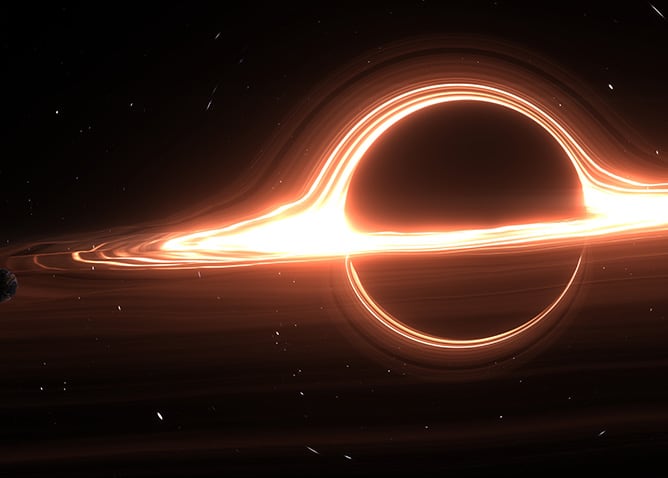
Black holes, neutron stars, or white dwarfs --- these remnant objects which remain after the death of stars are called "compact objects". These are often associated with plasma flows attracted by their strong gravity. These swirling plasma flows are called accretion disks or accretion flows. They are natural probes for us to observe the spacetime structure under strong gravity, and X-ray astronomy has investigated them significantly. One of the most important results is the iron emission line profile observed by ASCA satellite. The iron line profile is distorted not only by the high speed rotation of accretion disk, but also by the gravitational redshift caused by the central black hole. Follow-up investigations showed that the accreting flow is not simple, but consists of various structures of plasma of different density or temperature, varying in time. In order to distinguish these structures and understand the nature of accretion flows and distorted spacetime, we need the precision X-ray spectroscopy provided by XRISM.
- Home
- Missions
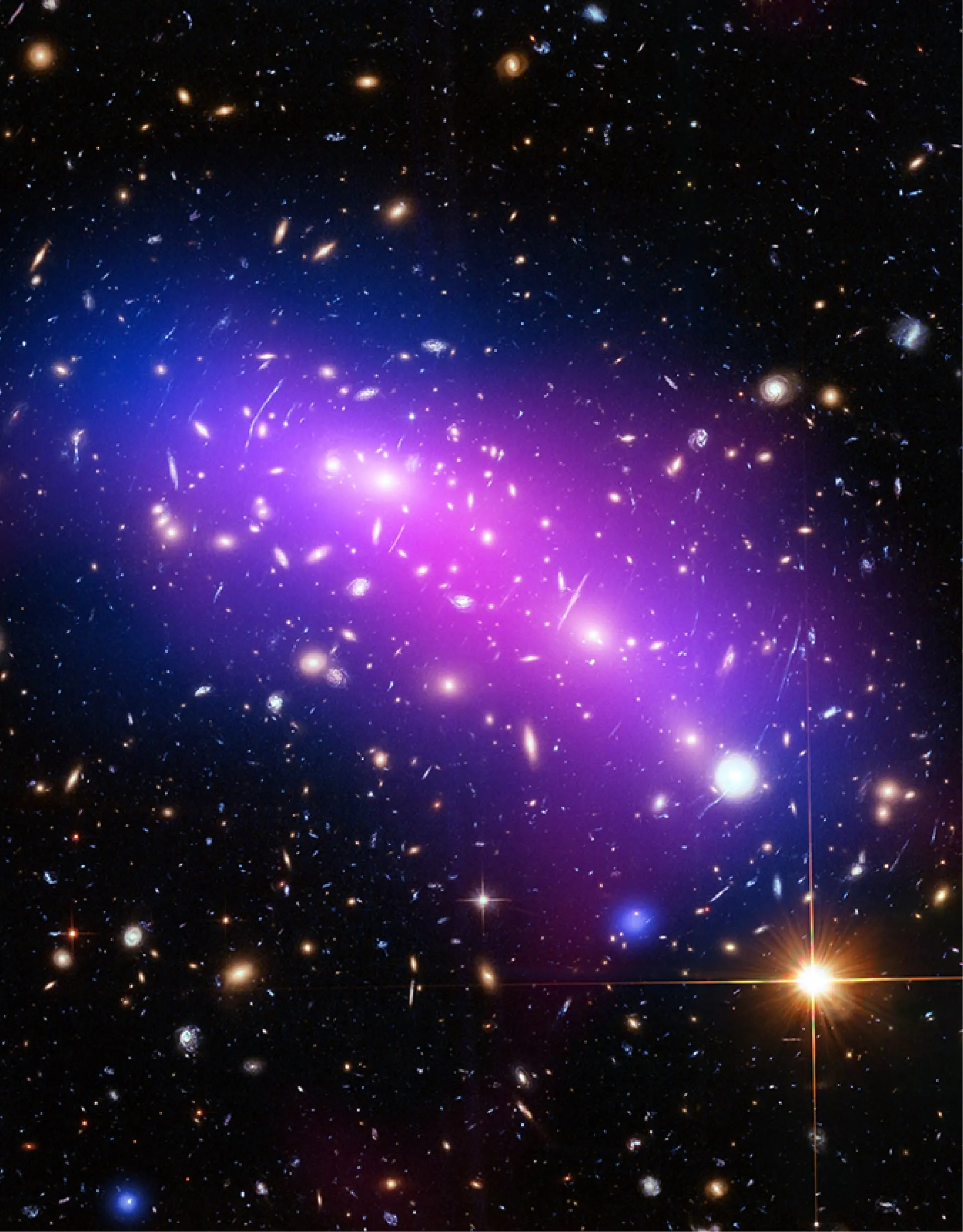